The material on this page is part of Chapter 10, which is shown in full as a preview on this site.
Chapter 10: Nucleic Acid Platform Technologies
Rando Oliver, Department of Biochemistry and Molecular Pharmacology, University of Massachusetts Medical School, Worcester, Massachusetts 01605
Although the majority of early microarray studies focused on mRNA expression profiling, microarrays can be used for any purpose that involves comparison of two nucleotide populationsfor example, comparison of RNAs extracted from normal and tumor cells. In this section, we list several examples (by no means exhaustive) of published microarray analyses.
Early gene expression microarrays consisted of arrayed spots each containing a cDNA corresponding to a single gene. For example, one of the first yeast gene expression microarrays consisted of about 6000 spots composed of cDNA corresponding to nearly every gene in the Saccharomyces cerevisiae genome (DeRisi et al. 1997). cDNA microarrays have fallen into disuse because of the advent of oligonucleotide microarrays. Advances in oligonucleotide synthesis make it possible to generate gene expression microarrays composed of long oligonucleotides that have been designed to be complementary to a given gene. Oligonucleotides can be synthesized and sold in soluble form, which are then arrayed by a spotting robot, as with cDNA arrays. Alternatively, several commercial microarray suppliers use in situ oligonucleotide synthesis, in which oligonucleotides are printed directly onto the microarray substrate. This process allows for much more flexible generation of oligonucleotide microarrays and, thus, is used more often than soluble oligonucleotide arrays because a laboratory need not purchase an excess of oligonucleotides in order to run microarray experiments.
A typical mRNA expression experiment begins with the isolation of poly(A) RNA from two related populations of cells, such as yeast growing at 30C and yeast that have been shifted from 30C to 37C. Fluorophore-labeled cDNA is prepared from each mRNA population. Typically, the cyanine dyes used are Cy5 (pseudo-colored red in microarray images) and Cy3 (pseudo-colored green). The labeled cDNAs are mixed and hybridized to a microarray. After hybridization and washing, the microarray is scanned with lasers that excite the fluorophores, and the image is processed, generating a Cy5/Cy3 ratio for every spot on the array. The microarray data are normalized, and a list of the Cy5/Cy3 ratios per gene is generated, which can be analyzed for genome-wide changes in the transcriptome.
The microarray data are normalized so that the average log2(Cy5/Cy3) is 0; this is a necessary step because the absolute intensity of either channel (Cy5 or Cy3) is subject to a wide range of modifying influences, such as input RNA level and labeling efficiency, among others. This normalization should always be kept in mind in interpreting microarray studies. For example, microarray results for a comparison between liver RNA and sperm RNA populations might indicate that a number of RNAs are relatively enriched in sperm, but the absolute numbers of those RNAs per nucleus are still probably lower in sperm than in liver given the disparity in RNA abundance in the two types of cells.
The experiment described above is capable of providing information about the relative abundances of every mRNA expressed in the two cell populations. What about absolute expression levels? These can be assayed in two ways. First, single-color experiments using the Affymetrix platform provide a reasonable measure of mRNA abundancethe intensity of a given spot is related to the absolute abundance of its complementary mRNA in the original labeled population. Second, for two-color experiments, a straightforward way to estimate transcript abundance is to compare labeled mRNA with labeled genomic DNA, which, in principle, should be present in a single copy per gene (unless the gene in question comes from a multicopy family). Thus, gDNA is labeled with Cy3, mRNA is labeled with Cy5, and Cy5/Cy3 ratios provide a relative measure of mRNA abundance. These ratios can be converted to absolute mRNA abundance if the absolute abundance of any RNA is already known. Normalization can be used to set the absolute level of these RNAs, and all remaining RNA abundances can be inferred from their Cy5/Cy3 ratios relative to the Cy5/Cy3 ratios for the known standards.
Comparative genomic hybridization (CGH) is used to detect changes in DNA copy number across a genome (Pollack et al. 1999). In a CGH experiment, the two populations of genomic DNA are fluorescently labeled using Klenow DNA polymerase. Labeled DNA is compared using the same experimental design as for analysis of mRNA expression. Changes in copy number are reflected in the Cy5/Cy3 ratios. This method has been used most commonly in the study of tumors, where there is a well-documented role both for gene loss and for amplification in the process of oncogenesis. By analyzing changes in gene copy number, regional amplification or even whole chromosome copy-number changes have been characterized. Variants of this approach have also been used to characterize replication timing, as DNA isolated during S phase shows variations in copy number, based on whether or not the segment of genomic DNA has replicated.
Although changes in mRNA levels can be analyzed easily using microarrays constructed with one probe (spot) per gene, different methods are required to detect variations in transcript structure. These variations include changes in splicing patterns and in the start and stop sites for transcription. These and other changes in transcript structure can be detected and mapped using tiling microarrays. For example, transcriptional start sites can be mapped to 1020-nucleotide resolution by a variant of the absolute abundance hybridization method described above. Briefly, mRNA is labeled with Cy5 and hybridized against Cy3-labeled genomic DNA. In principle, an expressed RNA should appear as a long square wave of high Cy5/Cy3 ratios: Cy5/Cy3 ratios should be low in spots corresponding to genomic sequences that flank those complementary to the RNA. High Cy5/Cy3 ratios should start at spots containing sequences corresponding to the 5 end of the RNA and should continue to the end of the RNA (Fig. 2). Indeed, this technique has been used to determine transcriptional start sites to 20-nucleotide resolution in yeast (Yuan et al. 2005) and to detect and map novel transcripts in human cells (Shoemaker et al. 2001).
Variations in splicing patterns can also be detected and mapped using microarrays. With tiling arrays, expressed exons should appear as peaks of Cy5/Cy3. Another way to determine exon inclusion is to use an exon microarray, in which each spot on the array corresponds to a single exon. When probing an exon microarray, for a given tissue all of a gene's expressed exons will show high Cy5/Cy3 (Shoemaker et al. 2001).
Tiling microarrays have also been used to discover noncoding RNAs and novel genes. The reason for this is obviousa classical, expression microarray only covers what is known, whereas a tiling microarray can be designed to contain an entire genome. For example, tiling microarrays of chromosomes 21 and 22 revealed the nearly pervasive low-level transcription of the human genome (Kapranov et al. 2002).
RNA molecules associated with specific RNA-binding proteins have been identified using immunoprecipitation assays to isolate a protein of interest. The associated RNA molecules (or regions of RNA) are then compared with nonspecific RNAs that have been isolated in control immunoprecipitations lacking antibody (Hieronymus and Silver 2003; Gerber et al. 2004).
The functional properties of RNAs (e.g., translation) can be assayed by microarray using more sophisticated fractionation techniques. In an early study, polysomal RNAs were analyzed by microarray techniques (Arava et al. 2003). Subcellular localization of RNAs can also be studied when appropriate purification techniques exist; for example, isolation of dendrites from neurons has been used to identify the relevant RNA populations (Eberwine et al. 2002).
At present, one of the most popular applications of tiling microarrays is known as chromatin immunoprecipitation on chip (or ChIP-on-chip; see Chapter 20). By this method, the location of a specific protein, such as a transcription factor, is assayed through the use of chromatin immunoprecipitation. Briefly, proteins are covalently cross-linked to the genome using formaldehyde, and chromatin is sheared by sonication to small (500 bp) fragments. Using an antibody specific to a given protein (with either an antibody to an epitope tag, a protein-specific antibody, or even an antibody against a specific modification state), DNA associated with the protein in question is isolated by immunoprecipitation. After washing, the cross-links are dismantled and genomic DNA is isolated. The DNA is typically amplified, and the amplified material is labeled and hybridized competitively against labeled amplification reactions from no-antibody controls or from pre-IP material. Analysis of ChIP-on-chip microarray results provides a snapshot of a transcription factor's location on the genome and has proven to be a tremendously powerful method for studying transcriptional regulation by transcription factors and chromatin (Ren et al. 2000; Iyer et al. 2001).
Tiling microarrays can also be hybridized with genomic DNA that has been treated diagnostically with a nuclease before labeling the DNA. This method takes advantage of the fact that chromatin packaging of the genome dramatically affects nuclease accessibility. Two nucleases have been used in genome-wide studies. First, DNase Ihypersensitive sites have long been known to occur at genomic regulatory elements such as promoters, enhancers, and insulators. Here, chromatin is lightly digested with DNase I, sites of cleavage are isolated, and DNA surrounding cleavage sites is interrogated by tiling microarray analysis (Sabo et al. 2006). Second, micrococcal nuclease (MNase) is a nonprocessive nuclease with preference for the linker DNA between nucleosomes. Analysis of the DNA protected from MNase cleavage including comparison to whole genomic DNA has proven to be an invaluable tool in genome-wide nucleosome mapping studies (Yuan et al. 2005).
Although exon microarrays (see above) can monitor expression of each exon represented on the array, the structure of the transcript cannot be discerned. Specifically, exon connectivity is not detected using exon microarrays. For example, expression of exons 1, 2, 3, and 5 could indicate a single species containing all four exons, or two or more distinct species (1-2-5 and 1-3-5, etc.). To determine connectivity of exons requires the use of splicing microarrays, which are comprised of oligonucleotides complementary to specific splice junctions.
The sequence specificity of DNA hybridization has also been leveraged for genome analyses such as resequencing and polymorphism detection. In these applications, each genomic location is represented on the microarray by several oligonucleotides that differ by a single nucleotide. In other words, if the genomic region of interest has the sequence AATGCCA, oligonucleotides containing AATTCCA, AATCCCA, and AATACCA will also be printed. Hybridization of genomic DNA to these oligonucleotides can be used to determine mutations or sequence polymorphisms at the site interrogated by the set of oligonucleotides.
One way to use microarrays for resequencing is the so-called sequence capture protocol (Hodges et al. 2007). A microarray is printed with oligonucleotides corresponding to genomic regions of interest. DNA or RNA is hybridized to the microarray, and all complementary sequences are retained on the microarray through the washing protocols. After retention of desired genomic regions via hybridization, this hybridized material is eluted and sequenced using high-depth sequencing methods (Chapter 11).
Chapter 10 Protocols:
- Protocol 1: Printing Microarrays
- Protocol 2: Round A/Round B Amplification of DNA
- Protocol 3: T7 Linear Amplification of DNA (TLAD) for Nucleosomal and Other DNA < 500 bp
- Protocol 4: Amplification of RNA
- Protocol 5: Direct Cyanine-dUTP Labeling of RNA
- Protocol 6: Indirect Aminoallyl-dUTP Labeling of RNA
- Protocol 7: Cyanine-dCTP Labeling of DNA Using Klenow
- Protocol 8: Indirect Labeling of DNA
- Protocol 9: Blocking Polylysines on Homemade Microarrays
- Protocol 10: Hybridization to Homemade Microarrays
Now Available in eBook Format!
Get the eBook for 25% off or Bundle with Print for Extra Savings.(Limited time special offer.)
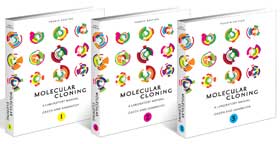
Search for information about other protocols included in the book:
Read What Others Are Saying About Molecular Cloning:
* Free shipping to individuals in U.S. and Canada only